When Mckenna Kleinmaier MAT ’20 teaches her environmental science class at Alexander Hamilton High School in Los Angeles, she dives right into challenging and complex topics: A recent discussion included how the human population is connected to global poverty and hunger. “We looked at demography, and the students can see very clearly there are issues—and they’re coming up with their own solutions,” Kleinmaier explains. In her classes, there is a focus on doing, not just repeating what the teacher says. “We are practicing developing models, constructing explanations: This is the future of science education.”
Kleinmaier, a Knowles fellow, is part of a movement of science teachers seeking to reinvigorate the field. The stakes are high: Even as women and minorities have increasingly entered careers in science, technology, engineering and math since 1990, they are still underrepresented overall. In the United States, kids are three times more likely to say they want to be a YouTube star than to be an astronaut when they grow up. This, coupled with an increasing distrust of science that has manifested itself in the fear of vaccinations and a reluctance to accept the dangers of COVID-19, has made it acutely necessary for there to be changes in how science is taught in schools. At USC Rossier, two innovations are taking hold: making students into scientists themselves, and increasing access to STEM for everyone.
Teaching science by doing science
For Kleinmaier, teaching science means STEM becomes an action word: Scientists are doers. “It’s not watching the teacher do it and doing the same exact thing,” she says. She tries to build curiosity in her students by looking at relatable phenomena. For example, it rained this morning—but now it’s sunny. “So a teacher might say, OK, I can give you the steps of the water cycle,” she explains. But instead, she tells her students to be doers of science. It rained, but the rain is gone off the sidewalk. Why did it start, where did it go? “From there, you can start to explore and investigate that phenomenon by running experiments, building models and constructing explanations. It naturally encourages curiosity and confidence in their learning.”
Following students’ questions can lead to a cascade of new lines of inquiry—and the doing of science does not need to be limited to students. Associate Professor of Clinical Education Angela Laila Hasan’s recent research focus involves looking at the cross section of family, teacher and student engagement in the experiential learning of mathematics. Hasan, who started her career as a middle school math and science teacher, has found in her research and teaching experience that parent engagement is one ticket to keeping kids interested and engaged in challenging topics.
It doesn’t mean that parents know as much as the teacher knows, but engaging them in experiential learning in STEM has direct benefits. Hasan watched as parents would do a physics experiment themselves, charting the path of a car down a ramp. The excitement of the parents was palpable to their kids. “I learned that if the family values what I am doing, it is more likely that the child is going to fall in line,” Hasan says. “Parent involvement is just the key to so much potential change. When we leave them out of the equation, as opposed to engaged in the process, we’re doing a disservice—and it does not take degrees in order to value science.”
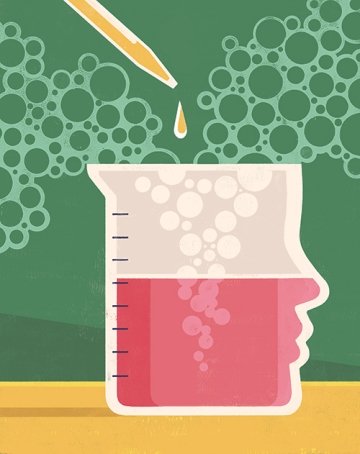
Professor of Clinical Education Frederick W. Freking, who taught high school science and eventually combined his research background with science teaching instruction, says there are some straightforward improvements to science education that teachers tend not to do—like keeping kids talking to each other, he says. “The amount of time is just not what it should be in terms of teacher talk versus student talk in the classroom,” he says, “Sharing ideas is a key part of science learning.”
It all has to do with how science is something that is done actively, not something to be passively acquired. “How can you be creative when all you do is sit there and listen?” Freking asks. “As a scientist, they need to ask questions, come up with ways to collect data and answer those questions.” Freking’s focus is on elementary teachers, who instruct a little bit in science but also have to teach language arts, social studies and math—the whole gamut. He works on something called the “5E” model of instruction: engage, explore, explain, elaborate, evaluate. The method isn’t brand new: It came out in the early ’80s, but it helps teachers implement what they know is important.
He explains that within the framework, the STEM lesson always starts with an engaging activity—something cool to connect to students’ culture or what they care about. “It should be something that hooks them, and then let them ask questions about that phenomenon,” he says. “Then they’re exploring it, letting the data lead to the finding, not because the teacher told them something.”
Teachers can then layer in the academic language, coming from context and understanding. After that there’s an elaboration phase, which allows students to ask additional questions and do different experiments and then share with one another. “It really helps kids see what science really is: It’s a way of knowing, not just a list of facts,” Freking says, adding that teachers can take the 5E model to any context they want to teach. One of the ways that he helps teachers sharpen their STEM teaching skills is through video review: Just as a coach would do for a sports team, the teachers gather and watch each other’s actions on video, giving criticism and advice on how to improve.
For Anthony Maddox, a professor of clinical education and engineering, one way to approach STEM is to focus on the notion of practice—the practice of constructing an environment where learning takes place. “I try to co-construct the learning space with the students, so it’s learning-centered and not necessarily content-centered,” he says, adding that he wants students to know that he, as a teacher, is also learning. “One of the roles I have in my position is not to suggest that I am some fount of knowledge, but I am here learning and I mediate the learning.”
Often that means working in smaller groups, where students are talking more and experiencing more. “As human beings, we are experience-based in some kind of way, and we’re acknowledging the fact that student experience is important,” Maddox says. “I’m taking a bet: While they may drift off topic, while they are free to express, when they land on topic they will be engaged.”
“How can you be creative when all you do is sit there and listen? As a scientist, students need to ask questions, come up with ways to collect data and answer those questions.” —Frederick W. Freking, USC Rossier Professor of Clinical Education
When it comes to mathematics, Maddox endorses an approach that links math to other sets of symbols that describe or manipulate real things in the world—like computer code that creates software to schedule meetings, or a foreign language that lets you find a train going to your destination. “My perspective is that if you master a symbol set in the world, you have strong opportunities to do things you want to do,” he says. In his view, there are five symbol sets that can describe different parts of the world: natural or spoken languages, artificial languages like computer code, music, mathematics and currency. Deep knowledge of any of those sets of symbols gives a student access to good jobs and opportunities, he says. And all of these sets can be an original tool in the hands of students making creative decisions—moving currency around, or composing a musical score.
Maddox tries to infuse every teaching endeavor with the six engineering habits of mind identified by the National Academy of Engineering: systems thinking, creativity, optimism, collaboration, communication and ethical consideration. “I can take any content and make sure that any of those six ideas prompt students to think about the content of that course, so for me that’s engineering,” he says. “Another view of engineering is leveraging technology for useful purposes. And that ties into teaching, because teachers leverage learning phenomena for useful purposes.”
He’s particularly excited about the fusion of education, engineering and entrepreneurship in hackathons and maker spaces—places where students really get to design their own ideas and make them come to life. But ultimately, his view of STEM education comes down to students’ own aspirations. “We shouldn’t lose sight—all these things should work together to create hope,” Maddox says. “I see every student as an individual who needs their hope reaffirmed. And my role is to give them the space and freedom to learn.”
Increasing access to STEM
For students to fully absorb all the benefits that STEM has to offer, they need to have access and encouragement to grab opportunities. Historically, minorities, girls and women have been excluded in STEM—but that is slowly changing.
Mentoring can be a key way for students to see themselves in future STEM careers, says Associate Professor of Education Darnell Cole, who co-directs USC Rossier’s Center for Education, Identity and Social Justice. He and the center’s co-director Shafiqa Ahmadi, associate professor of clinical education, are working to create a mentoring program called Black Seeds. The program would form mentoring relationships between professors and college students with middle schoolers—with a focus on underrepresented students. The hope is that these multilevel mentorships will build strong foundations for students to enter STEM fields in the future.
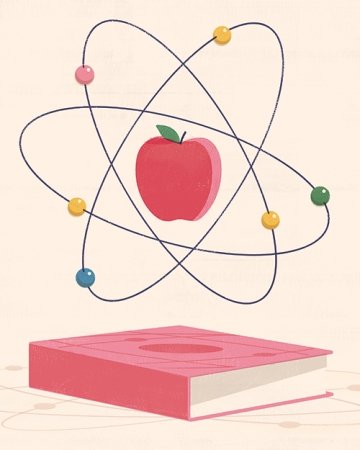
Why start so early in mentoring students? “Research shows that in order to cultivate interest toward college and cumulative-content subject areas like math and science, you have to start as far back as sixth grade for them to have courses they need to be competitive,” Cole says. “We also recognize that middle school is an incredible area of social-emotional development, and students develop the social capital in ways that are congruent with their community background.”
When students get fired up about STEM opportunities, they still need coursework that can support their advancement in specific content areas, like math and science. Expanding access to STEM classes and maintaining a high level of content may seem at odds—but it doesn’t have to be. Suneal Kolluri PhD ’19, an assistant professor at San Diego State University School of Teacher Education, has researched Advanced Placement tests. He says AP classes have been expanding rapidly with the goal of including students from marginalized backgrounds, to ensure that students are readying themselves for college.
Kolluri used data from the state of California on AP performance across the state, and looked at schools beating the odds. He focused on two schools succeeding at an unlikely task: increasing their AP access—so more were taking the courses, predominantly Latino/Latina students—and increasing their scores. He spent more than 100 hours at the schools, talking to students, watching classrooms, interviewing teachers and principals.
The schools were succeeding in very different ways. One school adopted a no-excuses mindset, where the principal believed strongly that all teachers could teach at a high level, and all kids could succeed in taking college-level classes.
“Cultural connections work in terms of getting students to engage with rigorous and complicated stuff. …We need to think within the system and change the system to allow for more access.” —Suneal Kolluri PhD ’19, San Diego State University School of Teacher Education Assistant Professor
The other school focused on being culturally relevant, communal and encouraging to students to explore cultural strengths through the curriculum. Those students also did exceedingly well, increasing access and performance. For example, a biology teacher centered her instruction on folk medicine in Mexican culture, and how many traditional healing methods were actually grounded in biology. “I think those connections work in terms of getting students to engage with rigorous and complicated stuff,” Kolluri says. “We need to rethink and reframe and re-envision because what we have been doing has not eliminated the opportunity gaps. We need to think within the system and change the system to allow for more access.”
In K–12 and higher education, teaching science goes a lot deeper than just creating future scientists, says Gale Sinatra, the Stephen H. Crocker Professor of Education. It’s to have a future architect think scientifically and critically about what type of building to create, or why an artist would use a certain type of paint mixture, she says. And the critical thinking and questioning that happens in these foundational years will follow through to help the students of today tackle all kinds of misinformation—from climate skepticism to unbased vaccine fears. “Scientific thinking and reasoning permeates every field,” she says.